BY PEET VAN BILJON
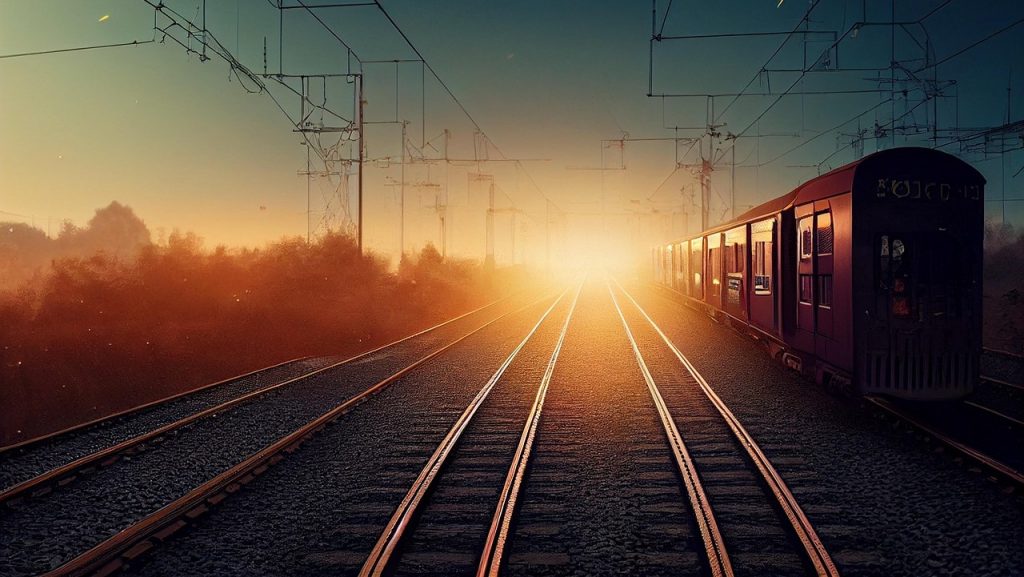
In uncertain times, the R&D portfolio is vulnerable to budget cuts. Tying each R&D project to a Business Impact that is justifiable at the Board level protects the R&D portfolio from capricious budgets cuts and provides a rationale for reallocating R&D resources when priorities change.
Why CTOs (Rightly) Hate NPVs
CTOs are often frustrated when asked to justify an R&D project based on its Net Present Value (NPV) or Return on Investment (ROI). Calculating these values for R&D projects are not feasible other than for late-state development projects that are close to market launch. A real danger of using NPV or ROI thresholds to determine which R&D projects make the cut is that early-stage research projects – for which NPV and ROI are impossible to calculate – will be disadvantaged relative to late-stage development projects. This may or may not be a desirable outcome in a particular strategic scenario, but such a bias risks leaving the portfolio empty of potentially important research needed to fuel the next business growth phase.
The fundamental problem with using NPV and ROI measures for R&D evaluation is twofold: First, they attempt to quantify into monetary units outcomes that cannot yet be so quantified; Second, they intentionally collapse the time and money dimensions into a single measure (respectively time-discounted dollars or percentage return) intended to also reflect the time value of money.
While “What is the NPV or ROI?” is the wrong question to ask for an R&D project, the intent behind the question is entirely legitimate, which is to gauge whether the R&D will add real value to the business. A better question is therefore how the R&D will impact key areas of the business. Reframing the conversation to be about the expected Business Impact, which is the contribution of R&D to important business outcomes, will facilitate more productive discussions between the CTO, the CEO and other executives, and the Board.
The goal of R&D portfolio management is to ensure that limited financial and human resources are deployed in service of the corporate strategy. The composition of the R&D portfolio is the ultimate responsibility of the CTO while the corporate strategy is the responsibility of the CEO, acting in concert with the Board. Considering the potential Business Impact of any R&D project or project proposal creates a built-in mechanism to align the R&D portfolio with the corporate strategy, and by implication to adjust the R&D portfolio as needed when the corporate strategy changes.
Constructing a 3D-Portfolio of R&D Projects
There are at least three major dimensions according to which each project in the R&D portfolio should be classified. I call this creating a 3D-Portfolio of R&D Projects. The three essential dimensions are
- the type or area of Business Impact;
- the relative magnitude of the expected Business Impact;
- and the approximate time horizon over which this impact is expected to happen.
Other dimensions or considerations may be added, such as the type of technology (technology family or generation), the level of maturity of the technology (though this will be highly correlated with the time horizon in most cases), or the extent to which an R&D project departs from current technology (incremental or radical change).
A sound R&D portfolio-management process will require making design decisions about each of these three dimensions, and then designing the process flows by means of which projects will be added to or removed from the portfolio. Typically, there will need to be a cyclical process (such as the annual budget cycle) and an exception-based process for when portfolio adjustments need to be made inflight.
The sections that follow provide guidance on designing the three dimensions, followed by a very brief discussion of designing the supporting portfolio management processes, as such processes will always be highly specific to any company or organization.
Step 1: Defining Your Set of Business Impact Areas
What is a suitable Business Impact to associate with an R&D project? A Business Impact must be more specific than “increasing revenue” or “lowering costs” or “making operations more sustainable.” However, it is the highest-level answer as to how specifically R&D can contribute to such top-level corporate goals. As such, each Business Impact is a vital link connecting desired R&D outcomes to corporate strategic goals.
Business Impact areas can be divided into three categories: those that are revenue-related, those that are cost-related, and a third category covering other areas such as sustainability-, safety-, or regulatory-related Business Impacts. It is advisable to employ a set of Business Impacts that includes at least one selected from each of these three categories.
While business objectives are best phrased at a high level, they also require sufficient specificity. This suggests looking to typical industry metrics or key performance indicators (KPIs) for inspiration. For example, a retail store chain may have the revenue goal “to increase sales per square foot” rather than simply to increase total revenue. An automotive OEM may have the cost-related goal of reducing “warranty cost per vehicle.” A chemicals company may have a KPI related to regulatory or environmental compliance, specified in terms of minimizing the “number of regulatory violations”.
Each industry has such KPIs with which everyone will be familiar. Table 1 contains examples for typical industries but is far from exhaustive. It is best to select about four or five such Business Impact areas in total for classifying your company’s R&D projects. If these have already been spelled out in a Board directive to the executive you are lucky, but absent that it is usually not hard to pick an uncontroversial set of business outcomes that R&D can impact and which few would disagree with.
The beauty of being able to classify each R&D project by the Business Impact dimension is that it makes it easy to answer questions from the Board such as “How much of our $50 million R&D spending is going to greenhouse gas (GHG) reduction?” In this example, if GHG reduction were one of your Business Impact areas (as it should be if it’s important for your company and in your industry), you would have the subtotal of R&D spending on that Business Impact readily available.
When the corporate strategy then needs to be adjusted due to say economic headwinds, the conversation can be about how to shift the balance of the R&D portfolio between Business Impacts. For example, the current R&D portfolio may be 30 percent allocated to a cost-related KPI such as “overhead ratio” in financial services. If a banking crisis causes the Board to demand a greater emphasis on such projects, it would mean shifting the balance to say 50 percent of the total R&D portfolio. Such a change may be achieved by increasing the number of projects related to that impact area, or reducing other projects, or by a combination of both actions.
Table 1. Examples of Industry KPIs Associated with Business Impact (Illustrative, Not Exhaustive)
Industry | Revenue-related (topline) | Cost-related (bottom line) | Other metrics |
Automotive | Market share | Warranty cost | Fuel efficiency; platform commonality |
Chemicals | Plant utilization | Energy usage per production unit | Number of regulatory violations; environmental compliance |
Consumer packaged goods | Average consumer spending (on company products) | Supply chain efficiency | Brand loyalty; percent of ethically sourced products |
Financial services | Assets under management | Overhead ratio | Risk-weighted assets; Basel III ratios |
Manufacturing | Capacity utilization rate | Scrap rate | Backorder rate; changeover time |
Metals & mining | Production volume | Cost per ton | Water quality index; safety |
Oil & gas | Break-even oil price | Lifting costs | Exploration success rate; carbon intensity |
Pharmaceuticals | Percentage of revenue from blockbuster drugs | Cost per new drug development | Pipeline strength; number of clinical trial failures |
Retail | Sales per square foot | Cost per square foot | Average discount depth; customer satisfaction score (CSAT) |
Technology | License and subscription renewal rates | Cost per line of code | Platform uptime; data breach incidents |
Step 2: Defining the Range Scale for Each Business Impact
How do you prioritize R&D proposals that are tied to different Business Impact areas? Moving away from a single metric such as NPV or ROI to compare a R&D project’s value-add to multiple Business Impacts means that you have to find a way of comparing apples with oranges. However, this is not as hard to do as it may seem but it does require a shift in mindset:
The common scale of comparison for all Business Impacts becomes an ordinal scale with three to four intuitive impact descriptions on it, such as Low, Moderate, High, and Very High. The design work is in defining what each impact description means for each Business Impact area. For example, if the Business Impact is reflected by a KPI that can typically only be moved in single digit percentages, it would look like the “Business Impact 1 KPI” in Table 2, where a “High” impact is classified as a 5 to 10 percent increase. Another Business Impact may be easier to inflect, such as “Business Impact 2 KPI”, and therefore may need to be improved by 20 to 30 percent for it to be considered a “High” impact.
Completing such a table for each Business Impact area versus the ordinal scale for the impact (e.g., low to very high) will result in an impact “rubric” which can be used to compare the potential relative benefit or value-add for R&D projects that are entirely different in kind, for example, an increase in market share versus engine fuel efficiency for an automobile OEM.
Table 2. Illustrative Rubric for Comparing Business Impact
Business Impact 1 KPI | Business Impact 2 KPI | |
Low | < 1% | < 10% |
Moderate | 1 to 5% | 10 to 20% |
High | 5 to 10% | 20 to 30% |
Very high | > 10% | > 30% |
Before launching the new rubric to grade the first R&D project proposals, the CTO’s team must take great care in calibrating the scales with the involvement of the entire R&D leadership and key stakeholders in other departments. That will create trust and prevent anyone from gaming the system to advance their pet projects.
It is also important to be fair and maintain the integrity of the grading for each R&D project by using peer review to check the claimed magnitude of the Business Impact KPI movement for each case under consideration. This is best done in an R&D budget decision meeting with all the major parties present.
Step 3: Defining the Time Horizons to Use
A multi-year classification framework is needed to indicate the approximate timeframe in which an R&D project’s Business Impact is expected to be achieved. The simplest and most intuitive approach is to use the Three Horizons, where Horizon 1 (H1) is the short term, Horizon 2 (H2) is the medium term, and Horizon 3 (H3) is the long term. For illustrative purposes, H1 is typically 12 to 18 months, H2 is 18 months to 3 or 4 years, and H3 is farther out. However, the durations will differ by industry as industries have shorter or longer cycle times. You’ll need to define the Time Horizons that make sense for your industry and apply them consistently.
The Time Horizons will facilitate the cross-referencing of the 3D R&D portfolio with your company’s Technology Strategy. For example, where your strategy is to only implement fairly mature technologies in a particular technology domain, that may result in only selecting R&D projects in that domain that fall within H1. On the other hand, if you see yourself as a technology leader in your industry for a particular domain, that would argue for having R&D projects all the way out into H3.
Creating the 3D-Portfolio of R&D Projects
By far the best way to have a portfolio of R&D projects that can each be tied to Business Impact is to create it that way from the start. This can be done by employing the portfolio framework described above.
Rather than simply inviting and collecting R&D proposals or ideas – as is often the case during the annual proposal cycle – you must give guidance on what types of proposals are desired and how they will be evaluated. You should map out the portfolio composition you want to end up with by constructing a table indicating desired allocations (percentages or dollars) by Business Impact versus Time Horizon. This guidance should be compiled ahead of the proposal invitation period by the CTO’s team in consultation with key business partners and with reference to the corporate strategic objectives for the year.
Following this process will effectively cascade the corporate strategic goals down to high-level R&D departmental goals and shape the resulting new R&D portfolio. [I previously described the complete hierarchy of innovation value-creation levels versus the parts of the organization responsible for each step in Chapter 7 of my book, Innovation for Value and Mission – An Introduction to Innovation Management and Policy.]
Once you have defined your 3D framework, you can use it to retroactively classify and organize all existing R&D projects. This may be necessary in case of a mid-cycle event such as receiving a new strategic directive from the Board or CEO that necessitates a rebalancing of the R&D portfolio. Having an R&D portfolio classified by the three dimensions will facilitate the most rational and unbiased way of making portfolio changes, even if there has to be cuts. And it will minimize the likelihood of making cuts that you’ll deeply regret later!
This article was originally posted by the author on LinkedIn.
©2025 Peet van Biljon. All rights reserved.